Why Healthy Individuals May Have an Elevated Hemoglobin A1c
Introduction
Hemoglobin A1c (HbA1c) is the most commonly used tool for the diagnosis, management, and monitoring of long-term blood glucose control and diabetes. Briefly, Hemoglobin A1c is a laboratory test that quantifies the amount of sugar that is bonded to red blood cells circulating in the human body. Specifically, it measures the amount of glucose linked to the protein hemoglobin through a process known as glycation. Hemoglobin A1c, often abbreviated HbA1c, provides an estimate of the average blood glucose concentration over a 10 to 12 week period. Abnormalities in Hemoglobin A1c can suggest the presence of insulin resistance, which contributes to many illnesses including cardiovascular disease, certain cancers, dementia, infertility, kidney and liver disease.
Importantly, however, there are several biological factors that may influence the reported value of Hemoglobin A1c, for which HbA1c may not be a reliable or accurate test in all subjects. Specifically, there are several recognized instances where Hemoglobin A1c is elevated in the absence of insulin resistance or blood glucose dysregulation. In other words, there are several distinct mechanisms where a seemingly healthy individual may have a borderline elevated or mildly abnormal Hemoglobin A1c value, occurring in the absence of blood glucose dysregulation or insulin resistance. Reassuringly, it appears that there are multiple benign and/or easily treatable circumstances that may contribute to a mildly elevated Hemoglobin A1c in healthy individuals without insulin resistance.
The intention of this post is to explore the physiology and evidence regarding elevated levels of Hemoglobin A1c in healthy individuals for reasons other than insulin resistance. This includes normal variations in red blood cell lifespan, as well as the concept of “exercise-induced insulin resistance,” for which a trained individual will prioritize fatty acids as a fuel source, while preserving glucose for future energy storage and utilization. This appears to be a protective mechanism that demonstrates characteristics of “exercise-induced insulin resistance.” Fortunately, this does not appear to be harmful or pathological cause for concern. However, this phenomena may contribute to elevations in Hemoglobin A1c among endurance athletes.
Importantly, a benign explanation for an elevated Hemoglobin A1c should be regarded as a “diagnosis of exclusion,” for which the evaluation of several pathological explanations should be identified and “ruled out” first. Therefore, this post will also provide a brief discussion regarding the most common pathological explanations of an elevated Hemoglobin A1c, as well as the limitations of Hemoglobin A1c.
The approach to understanding an elevated Hemoglobin A1c should first begin with an understanding of the most common and concerning causes of an elevated Hemoglobin A1c (Part I), an understanding of benign and physiological explanation of an elevated Hemoglobin A1c (Part II), and the limitations of Hemoglobin A1c (Part III).
Related Podcast Episode
Disclaimer
This content is for general educational purposes only and does not represent medical advice or the practice of medicine. The information in this post is not intended to aid in the prevention, diagnosis, or treatment of medical illness. Furthermore, no patient relationship is formed. Please discuss with your healthcare provider before making any dietary, lifestyle, or pharmacotherapy changes. Furthermore, I have no financial conflicts of interest to report or affiliations with any diagnostic testing companies mentioned in this post.
Notify Me of New Content
Provide your email address to receive notifications of new blog posts and podcast episodes.
Content Summary
The Basics of Hemoglobin A1c
Hemoglobin A1c is a commonly used blood test easily obtained from a single venous blood sample. The measured value is reported as a percentage of circulating hemoglobin molecules that have glucose attached through a process known as glycation. Glycation refers to the chemical reaction in which glucose binds to protein, including the hemoglobin, a fundamental protein of circulating red blood cells. Notably, glycation is an irreversible process. When blood glucose levels are high (hyperglycemia), glucose molecules attach to the hemoglobin in red blood cells. Prolonged episodes of hyperglycemia contribute to higher exposure of hemlogin to glucose, and thus, a higher proportion of glycated hemoglobin.1 Because of the typical lifespan of a red blood cell is approximately 120 days, Hemoglobin A1c is regarded as a useful measurement of the average blood glucose control over a 10 to 12 week period.
Notably, there are a variety of biological and physiologic factors that may influence the functionality, lifespan, and structure of a red blood cell and its constituent proteins. Consequently, these factors may also influence the reported value of Hemoglobin A1c, for which the reported value may not be reliable or accurate in all test subjects. Specifically, there may be instances where a Hemoglobin A1c is falsely elevated for reasons not relevant to insulin resistance. Meanwhile, the opposite is also true, in which some pathological circumstances and medicines/supplements may contribute to an inaccurately low Hemoglobin A1c (not discussed today). The emphasis of this post will focus on the discussion of physiological and seemingly benign explanations in apparently healthy individuals without insulin resistance. Importantly, however, these benign explanations should be regarded as a “diagnosis of exclusion,” for which several pathological explanations of an elevated Hemoglobin A1c should be evaluated and ruled out before concluding the possibility of a benign explanation for a moderately elevated HbA1c.
Figure 1. American Diabetes Association categories for Hemoglobin A1c2

Table 1. Hemoglobin A1c and Estimated Average Glucose2
Hemoglobin A1c (%) | Estimated Average Glucose (mg/dL) | Estimated Average Glucose (mmol/L) |
6.0 | 126 | 7.0 |
7.0 | 154 | 8.6 |
8.0 | 183 | 10.1 |
9.0 | 212 | 11.8 |
10.0 | 240 | 13.4 |
Part I. Pathological Explanations of Elevated Hemoglobin A1c
In the event that a seemingly healthy individual identifies an elevated Hemoglobin A1c, several pathological explanations should be ruled out before concluding the possibility of a benign or physiologic explanation for the elevated HbA1c.
i. Insulin Resistance
The most common explanation of an elevated Hemoglobin A1c is blood glucose dysregulation in the context of insulin resistance. These abnormalities form the basis of Type 2 Diabetes and Metabolic Syndrome. Insulin resistance often occurs in the context of excess body fat, excess belly fat (visceral adiposity), elevated blood pressure (hypertension), elevated triglycerides, low HDL cholesterol, and elevated blood glucose (hyperglycemia). Therefore, in individuals with an elevated Hemoglobin A1c, a thorough evaluation of metabolic health is necessary (Table 1).
Notably, the first compensatory mechanism of the human body experiencing insulin resistance is to increase the release of circulating insulin. As a result, increased levels of circulating insulin (hyperinsulinemia) can maintain normal blood glucose values (Figure 1). Despite normal blood glucose control, hyperinsulinemia is a pathological condition and potent risk factor for numerous cardiovascular and non-cardiovascular illnesses. Therefore, a fasting insulin level can aid in the identification of insulin resistance, including early stages of insulin resistance not identified with Hemoglobin A1c, fasting blood glucose, or continuous glucose monitoring.
Interestingly, abnormalities of lipoproteins are often some of the earliest abnormalities seen in new onset insulin resistance. As a result, this has led to the development of the Lipoprotein Insulin Resistance Score (LPIR), which is a mathematical model that quantifies the degree of insulin resistance present based upon characteristics of lipoprotein density and quantity. Therefore, in individuals with an elevated Hemoglobin A1c and any of the above metabolic abnormalities (e.g. Metabolic Syndrome), it is reasonable to obtain an LPIR Score to further understand the presence or absence of insulin resistance. This can be used in conjunction with a fasting insulin, fasting glucose, and the HOMA-IR model.
Summary Statement:
Insulin Resistance typically occurs in individuals with poor metabolic health, for which elevated levels of insulin are often present, sometimes with or without abnormalities in blood glucose. Among individuals with an elevated Hemoglobin A1c and concern for insulin resistance, a thorough medical evaluation would include an assessment of body composition, blood pressure, lipid and lipoprotein analysis, fasting insulin levels, and an LPIR score.
Table 1. Common Metabolic Abnormalities Associated with Elevated HbA1c
Metabolic Abnormality | Considerations |
Body Composition (Visceral Adiposity) | DEXA Imaging, Waist-to-Hip Ratio, BMI |
High Blood Pressure (Hypertension) | Blood Pressure Assessment, 24-hour Blood Pressure Monitoring |
Lipids and Lipoproteins (Dyslipidemia) | Elevated Fasting Triglycerides, Low HDL-C |
Blood Glucose Dysregulation (Hyperglycemia) | Elevated Fasting, Prandial, Average Blood Glucose (Continuous Glucose Monitor) |
Elevated Liver Inflammation | AST, ALT and Abdominal Ultrasound if Visceral Adiposity Present |
Insulin Resistance | Fasting Insulin + Glucose (HOMA-IR), Lipoprotein Insulin Resistance Score (LPIR) |
Figure 1. Stages of Insulin Resistance
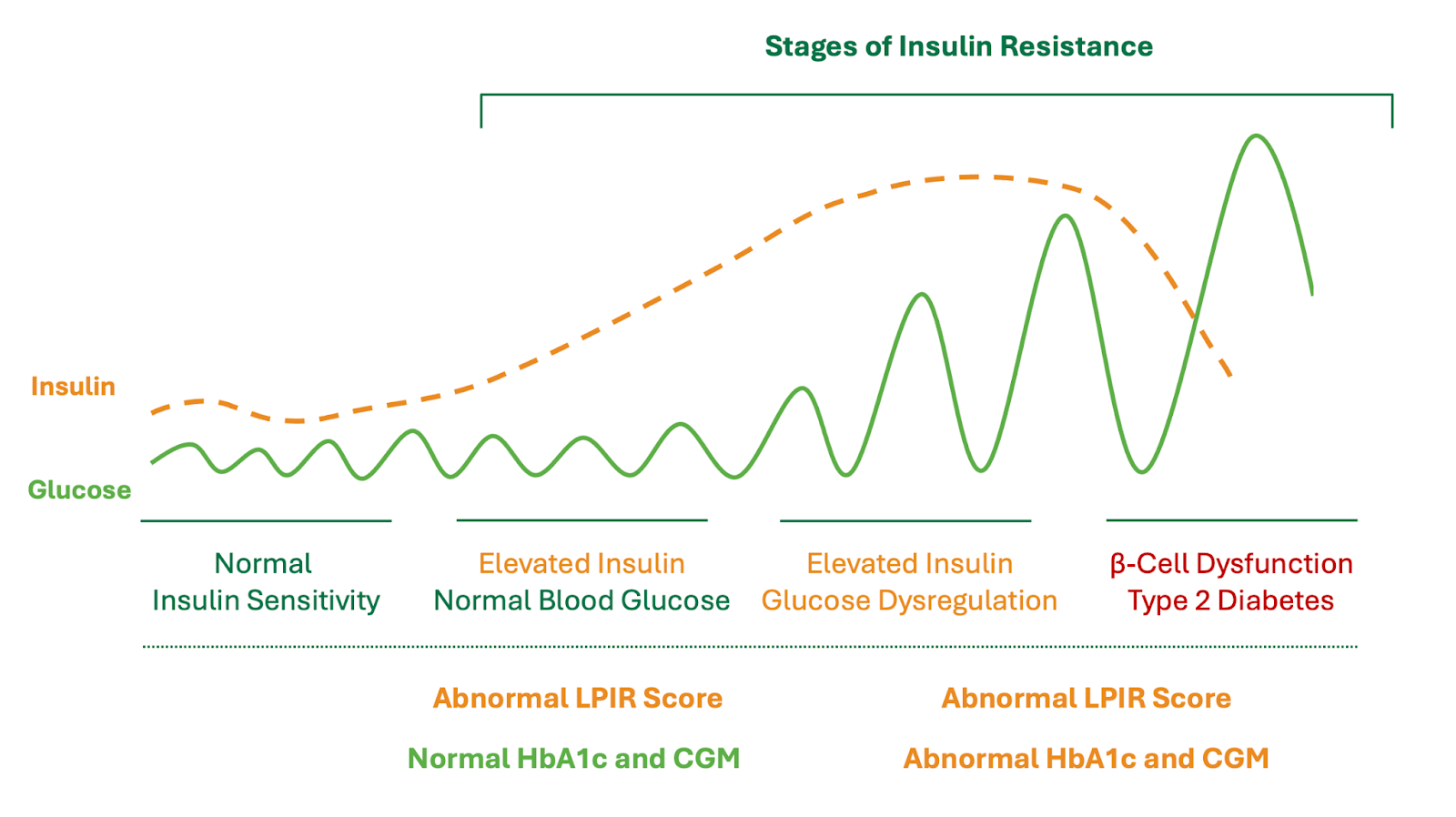
ii. Type 1 Diabetes
In the event that a seemingly healthy individual identifies the presence of an elevated Hemoglobin A1c and/or elevated blood glucose, one potential and consequential explanation is new onset Type 1 Diabetes. Briefly, Type 1 Diabetes is a distinct medical condition from Type 2 Diabetes, in which an autoimmune process contributes to the destruction and dysfunction of the insulin-producing beta cells in the pancreas, leading to a deficiency in insulin production. As a result, there is inadequate insulin produced by the body, resulting in persistently elevated blood glucose levels as well as an elevated Hemoglobin A1c.
Unfortunately, the majority of individuals with Type 1 Diabetes present with severe manifestations of metabolic illness including dehydration, severe acid/base (pH) imbalances, electrolyte abnormalities, dangerously high levels of circulating blood glucose, and often coma. While Type 1 Diabetes has historically presented in children and adolescents, there has been a noticeable increase in the number of adults newly diagnosed with Type 1 Diabetes. Therefore, a seemingly healthy individual with an elevated Hemoglobin A1c and/or blood glucose should be evaluated for insulin resistance (Pre-Diabets and Type 2 Diabetes), which is the most common explanation for an elevated HbA1c. Meanwhile, there should also be awareness and consideration of Type 1 Diabetes for healthy individuals with unexplained or unexpected elevations in HbA1c. This is even more relevant for individuals with a family history of Type 1 Diabetes and/or certain autoimmune illnesses.
The diagnosis of Type 1 Diabetes is outside the scope of this post, however, this condition is typically diagnosed as a result of a combination of abnormalities including hyperglycemia, inappropriately low levels of insulin and C-peptide, and +/- characteristic antibody testing (ICA, GAD, IA-2, IAA, and/or ZnT8 antibodies). Importantly, any individual with concern for Type 1 Diabetes should be evaluated promptly by a licensed healthcare professional.
Summary Statement:
Type 1 Diabetes can spontaneously occur in healthy adolescents and young adults, for which the inadequate production of insulin will result in abnormal elevations of blood glucose (fasting and non-fasting), low levels of insulin and C-peptide, and possible detection of known antibodies.
iii. Iron Deficiency and Other Micronutrient Deficiencies
Multiple studies have demonstrated that iron deficiency can result in elevated values of Hemoglobin A1c in non-diabetic individuals.3,4,5 Furthermore, it has also been demonstrated that meaningful reductions in Hemoglobin A1c can be achieved with iron supplementation in individuals with iron deficiency.3,5 In many instances, the average reduction in HbA1c can be more than 1.0%, for which some individuals may transcend categorizations of Pre-Diabetes and Diabetes into a Normal HbA1c range.
A potential physiologic explanation for this observation is based upon the recognition that iron deficiency contributes to a reduction in red blood cell (RBC) production. As a result, the body compensates by increasing the average lifespan of circulating red blood cells. Because (1) glycosylation of hemoglobin is an irreversible process, and (2) a longer RBC lifespan will contribute to the increased cumulative exposure of hemoglobin and circulating blood glucose, this may ultimately lead to elevation in HbA1. Importantly, this abnormality is due to the prolongation of average RBC lifespan, rather than blood glucose dysregulation or insulin resistance.6
There are several common causes of iron deficiency, some of which are particularly consequential (e.g. gastrointestinal cancer) and the identification of iron deficiency warrants a formal medical evaluation from a licensed healthcare professional (Table 2).
Table 2. Common Causes of Iron Deficiency
Common Causes | Example(s) |
Inadequate Dietary Intake | Restrictive Dietary Patterns |
Increased Iron Requirements | Pregnancy, Rapid Growth Periods (e.g. puberty) |
Gastrointestinal Malabsorption | Celiac Disease, Inflammatory Bowel Disease, Gastric Bypass |
Blood Loss | Gastrointestinal and Hemorrhoidal Bleeding, Gastrointestinal Cancer, Menstruation |
Chronic Infection | Parasitic Infection (e.g. Hookworm) |
Medications | Proton Pump Inhibitor, Antacids, Certain Antibiotics |
Magnesium deficiency has also been associated with insulin resistance through several mechanisms. Specifically, low magnesium can result in impairment of tyrosine kinase activity relevant to the function of insulin, glucose transport, and glucose utilization. In animal studies, for example, magnesium supplementation increased mRNA production of glucose transport proteins (GLUT4) by approximately 23%.7 Interestingly, persistently elevated levels of insulin have also been demonstrated to increase urinary excretion of magnesium, for which individuals with hyperinsulinemia and insulin resistance are at risk of magnesium depletion.8 Whether or not magnesium supplementation in magnesium-deplete individuals will result in meaningful improvements in HbA1c has not been convincingly established to the extent seen in the treatment of iron deficiency.
Additionally, chromium deficiency has been observed in individuals with insulin resistance, and some have suggested that chromium improves insulin sensitivity. Meanwhile, there are no high-quality experimental trials demonstrating a meaningful improvement in clinical outcomes relevant to insulin resistance through the chromium supplementation.9
Summary Statement:
Iron deficiency can contribute to elevated levels of Hemoglobin A1c that do not accurately reflect an individual’s blood glucose control or risk of insulin resistance. A potential explanation of this observation is that iron deficiency contributes to impairment in red blood cell production, for which the body compensates by prolonging red blood cell lifespan.6 Therefore, the average cumulative exposure of hemoglobin and circulating blood glucose is increased, which then increases the measured value of HbA1c for reasons unrelated to blood glucose dysregulation or insulin resistance. Notably, treatment of iron deficiency can improve values of HbA1c by more than 1%, enabling individuals to transcend pathological categories of Diabetes and Pre-Diabetes into a Normal Hb1c range.6 Meanwhile, iron deficiency can be a manifestation of serious underlying metabolic conditions that warrant a formal medical evaluation by a licensed healthcare professional (Table 2).
iv. Sleep Impairment
In addition to observational data suggesting that inadequate sleep duration is associated with insulin resistance,10-13 there is a growing body of experimental evidence demonstrating the negative health impact of sleep deprivation regarding insulin sensitivity and risk of insulin resistance.14,15
In a sample of 38 women without pre-existing insulin resistance, a randomized, crossover study with two 6-week phases was conducted, including a (1) maintenance phase of adequate sleep, and a (2) sleep reduction phase by 1.5 hours per night. The crossover study design implies that some participants were randomly assigned to begin with the sleep maintenance phase followed by the sleep reduction phase, and others were randomly assigned to begin with the sleep reduction phase followed by the sleep maintenance phase. The benefit of a crossover study design is that it allows for measured outcomes within a single participant, which increases the precision of identifying differences in health outcomes. In this study, it was demonstrated that fasting insulin levels were negatively impacted by sleep reduction, and this negative health outcome appeared more pronounced in post-menopausal women. Interestingly, there was no impact on fasting blood glucose values, again highlighting the limitations of monitoring blood glucose alone.
Summary Statement:
Sleep deprivation is a common but modifiable risk factor that has been demonstrated to impair insulin sensitivity and fasting insulin levels among healthy individuals. Experimental evidence in humans suggests that chronic sleep deprivation less than 7 hours per night can promote insulin resistance in otherwise healthy individuals.14
v. Persistent Stress and Inflammation
There is increasing awareness of the relationship between our psychological health and human physiology. Specifically, chronic persistent stress can negatively impact human health through a variety of distinct biochemical pathways. For example, under stressful life circumstances, the repeated activation of stress response pathways can contribute to the excess release of stress hormones such as cortisol and catecholamines, which can increase blood glucose levels by promoting gluconeogenesis (the production of glucose from non-carbohydrate sources), impairment of insulin signaling pathways, and a reduction in insulin sensitivity (greater insulin resistance).16 Elevated levels of cortisol can also promote the disproportionate tendency for the body to distribute new fat storage into the abdomen (fatty liver and visceral adiposity), which independently contribute to insulin resistance, cardiovascular disease, and numerous non-cardiovascular diseases.17,18 It has also been recognized that chronic stress and inflammation can lead to the release of pro-inflammatory signaling molecules (cytokines), including tumor necrosis factor-alpha (TNF-alpha), which is known to interfere with insulin signaling pathways and insulin sensitivity.19 As a result, it is recognized that chronic stress and inflammation can negatively impact insulin resistance and abnormalities in blood glucose control. In genetically susceptible individuals, this may contribute to measurable elevations in Hemoglobin A1c.
Aside from our psychological health, there are instances where chronic persistent inflammation may also negatively impact insulin sensitivity. Two common examples of chronic persistent inflammation include autoimmune illness and chronic infection. For example, among individuals with rheumatoid arthritis, a chronic autoimmune disorder characterized by inflammation of human joints, the chronic persistent state of inflammation can affect other organ systems, including our metabolism, endocrine system, and insulin sensitivity. To demonstrate this point, an analysis of more than 1.6 million individuals assessed the risk of diabetes among individuals with rheumatoid arthritis compared to the general population.20 It was demonstrated that rheumatoid arthritis was associated with a higher risk of diabetes (relative risk 1.23; 95% CI 1.07–1.40), suggesting that inflammatory pathways may be involved in the pathogenesis of insulin resistance and impaired insulin sensitivity.
Summary Statement:
It is recognized that chronic persistent stress and inflammation can contribute to insulin resistance through a variety of biochemical pathways. While there is enthusiasm for diagnostic testing among health conscious individuals and healthcare professionals, stress and inflammation are most accurately assessed by a thorough clinical evaluation from an experienced healthcare professional. With this information, additional measures of inflammation can be assessed with tests such as high-sensitivity C-reactive protein (hs-CRP), Homocysteine, Ferritin, and IL-6, however, these are highly non-specific tests and should be considered in the context of a thorough healthcare assessment of possible autoimmune, inflammatory, infectious, and metabolic illnesses.
Part II. Benign and Physiologic Explanations of an Elevated Hemoglobin A1c
Despite numerous pathological conditions that contribute to an elevated Hemoglobin A1c, it is increasingly recognized that there are healthy individuals with elevated levels of Hemoglobin A1c but no measurable degree of insulin resistance. In other words, there appear to be benign explanations and circumstances that may contribute to an elevated Hemoglobin A1c among certain individuals.
While the subject of an elevated Hemoglobin A1c in healthy individuals is an interesting subject to contemplate and discuss, it is not a well-studied field of research. The two most apparent explanations for this benign observation include natural variations in the lifespan of red blood cells in everyday individuals, as well as an elevated fasting blood glucose among some athletic individuals. As we will discuss below, these appear to be two possible explanations for why Hemoglobin A1c may be elevated in healthy individuals.
i. Prolonged Red Blood Cell Lifespan
As previously discussed, Hemoglobin A1c is a measure of the glycosylated hemoglobin as a result of circulating red blood cells encountering blood glucose. While HbA1c is most profoundly impacted by the concentration of circulating blood glucose, the average red blood cell lifespan is also an influential variable regarding the measurement of Hemoglobin A1c.
To demonstrate this point, researchers utilized a technique in which Biotin (Vitamin B7) was covalently attached to red blood cell proteins as a marker that could be measured with flow cytometry, ultimately providing insight regarding the lifespan of red blood cells.21 Importantly, both healthy individuals, as well as those with Type 1 and Type 2 diabetes, were evaluated. Notably, there was no meaningful difference in the lifespan of RBCs among healthy and diabetic patients. Rather, there was tremendous variation in RBC lifespan among all subjects. In other words, there appear to be natural variations in the typical RBC lifespan, irrespective of one’s health status. Specifically, in this study, substantial variability of RBC life span was observed, with mean RBC age ranging from 38.4 to 59.5 days, representing a 20% variation from the average. While it is possible that there are unidentified causes of this variation, it seems reasonable to conclude that much of this variability is due to genetic differences that are independent of dietary and lifestyle choices. Using a mathematical model to demonstrate the impact of RBC lifespan variability on HbA1c, these researchers demonstrated that an average RBC lifespan of 38.4 days may corresponded with a HbA1c of 2.4% lower than someone with an RBC lifespan of 59.5 days but identical blood glucose control. Importantly, these variations in HbA1c would impact risk stratification considerations and medical management.
Importantly, for interested readers, I am not aware of any commercially available test to assess or determine average red blood cell lifespan. Some physicians have acknowledged that a low Reticulocyte Count may imply a longer RBC lifespan. This concept derives from the understanding that Reticulocytes, or immature RBCs, are produced in response to low blood counts and the body’s desire to increase RBC production. When the body needs to increase its production of RBCs, the bone marrow releases more reticulocytes into the bloodstream, which can be measured by a Reticulocyte Count. Therefore, some physicians have suggested that a low Reticulocyte Count may imply a longer RBC lifespan, increasing the possibility that a measured Hemoglobin A1c may be elevated for reasons other than insulin resistance. While there is some logical basis in this train of thought, I am unable to find any relevant scientific research or literature suggesting that a low Reticulocyte Count correlates with a prolonged RBC lifespan (and potentially an elevated HbA1c).
ii. Physical Fitness, Impaired Glucose Utilization, and Exercise-Induced “Insulin Resistance”
There is no controversy regarding the positive health benefits of physical fitness, aerobic exercise, and endurance training. Interestingly, however, there appear to be several physiologic responses of the human body, for which there is evidence to suggest that regular aerobic exercise impairs glucose utilization and may increase Hemoglobin A1c. We will explore the data regarding these compensatory mechanisms and biological pathways.
Abnormalities in Hemoglobin A1c can result from elevations in (1) fasting blood glucose, (2) after-meal blood glucose, or (3) both fasting and after-meal blood glucose. Broadly speaking, it appears that abnormalities in after-meal blood glucose (prandial or post-prandial) are more specific to those with insulin resistance, whereas elevations in fasting blood glucose are observed in both endurance athletes as well as those with insulin resistance. To demonstrate this point, ten elite athletes were monitored with continuous glucose monitors (CGM) over a 6-day period.22 For context, these study participants were physically fit with a resting heart rate below 60 beats per minute, averaging more than six hours of physical fitness per week. Among the ten participants, 40% were observed to have a fasting blood glucose greater than 106 mg/dL (6.0 mmol/L) for more than 70% of the total monitoring time, even when excluding the 2-hour period after meals. Moreover, fasting blood glucose was in the pre-diabetes range for 30% of these athletes. Needless to say, these athletes did fit the traditional picture for someone with pre-diabetes or insulin resistance.
To help understand this paradoxical observation, it is necessary to review energy utilization during aerobic exercise, energy utilization following aerobic exercise, and hormonal adaptations among physically fit individuals. Experimental studies have demonstrated several favorable metabolic adaptations as a result of regular aerobic exercise. For example, trained athletes demonstrate lowering fasting insulin levels when compared to non-athletes.23 Furthermore, trained athletes appear to require less insulin to maintain similar glucose levels as non-trained individuals, demonstrating superior insulin sensitivity.24,25 While the basis of this post is a discussion of blood glucose control measured by Hemoglobin A1c, a far more important set of health metrics are fasting insulin, insulin resistance, and the importance of avoiding hyperinsulinemia. Therefore, despite possible increases in fasting blood glucose and/or Hemoglobin A1c in trained individuals, there is extensive evidence to suggest that aerobic exercise reduces fasting insulin levels and improves insulin sensitivity.
Next, the following study helps to reconcile why impaired blood glucose control and elevations in Hemoglobin A1c may be observed in trained athletes. In one highly informative study, nine endurance athletes and eight non-athletes were assessed under three conditions: (1) after an overnight fast without prior exercise, (2) after 3-hours of prolonged continuous exercise at 65% of VO2 max, and (3) 5×4 minutes of high-intensity interval training (95% of VO2 max).26 Oral glucose tolerance tests were performed in addition to an analysis of fasting free fatty acids, ketone levels, insulin sensitivity, glucose utilization, and fat utilization.
Remarkably, among the trained individuals, prolonged continuous aerobic exercise appeared to contribute to transient episodes of “insulin resistance” and impairment of glucose utilization. This observation was NOT observed in the non-athletes. Specifically, it appears that fatty acids are the prioritized fuel source for endurance athletes during episodes of prolonged aerobic exercise, as well as after prolonged aerobic exercise. While trained athletes demonstrated an increased ability to utilize lipids (fats) as a fuel source, the trained athlete’s body also demonstrated an adaptive response to reduce glucose uptake in a manner similar to “insulin resistance.” Importantly, many other studies have demonstrated the negative effect on glucose uptake after exercise.27-34 The authors of this study propose that the prioritization of utilizing free fatty acids as a fuel source, instead of circulating glucose, is an adaptive mechanism to maintain available glucose-energy for important organs such as the brain, as well as energy storage including the replenishment of glycogen for what the bodies future predicted high energy demands (i.e. the trained individual). The adaptation of “exercise-induced insulin resistance” functions as a protective mechanism to ensure efficient and reliable energy availability for the trained individual.
Consequently, it may be inferred that highly trained individuals may (1) maintain a superior level of insulin sensitivity while (2) prioritizing the oxidation and utilization of free fatty acids, which contribute to (3) higher than expected levels of fasting blood glucose. As a result, this may explain why some healthy, physically fit individuals demonstrate a higher-than-expected Hemoglobin A1c in the absence of insulin resistance.
Part III. Limitations of Hemoglobin A1c
Aside from the potential variations and inaccuracies of Hemoglobin A1c discussed above, it also recognized that Hemoglobin A1c does not adequately capture the potential risk of cardiovascular disease attributed to insulin resistance. Specifically, HbA1c is primarily a measure of long-term blood glucose control, where individuals with early stages of insulin resistance can still maintain normal blood glucose control, and thus, a normal HbA1c. The same limitations apply to continuous glucose monitoring and fasting blood glucose, which are measurements of short-term blood glucose control, rather than insulin resistance. The earliest manifestations of insulin resistance are characterized by elevated levels of insulin (hyperinsulinemia), which is a compensatory mechanism that allows the body to maintain normal levels of blood glucose during early stages of insulin resistance. In later stages of insulin resistance, blood glucose dysregulation can occur resulting in abnormalities of HbA1c, continuous glucose monitoring, and fasting blood glucose (Figure 1).
As discussed in a previous blog post, the Lipoprotein Insulin Resistance Score (LPIR) has emerged as a valuable tool to identify and quantify insulin resistance. Specifically, the LPIR Score has a unique ability to identify very early stages of insulin resistance, including those with normal blood glucose, normal HbA1c, and those with a normal body weight. Briefly, the LPIR Score utilizes NMR technology to measure lipoprotein abnormalities observed in insulin resistance, which is then reported as an LPIR Score from 0 (most insulin sensitive) to 100 (most insulin resistant). The clinical relevance of the LPIR Score has been demonstrated in large-scale prospective cohort studies, with evidence suggesting that it is one of the strongest predictive biomarkers of cardiovascular disease and future Type 2 Diabetes.35-38 Meanwhile, the LPIR Score can be obtained with a single fasting blood sample and it is relatively affordable.
Table 3. Comparison of LPIR Score to HbA1c as Predictors of Cardiovascular Disease35
ASCVD Before Age 55 | ASCVD Ages 55 – 65 | ASCVD Ages 65 – 75 | ASCVD Ages 75+ | No Development of ASCVD | |
LPIR score (0–100) | 65 (43 – 79) | 58 (35 – 74) | 55 (35 – 72) | 49 (28 – 68) | 39 (20 – 60) |
Hemoglobin A1c, % | 5.1 (4.9 – 5.4) | 5.1 (4.9 – 5.6) | 5.1 (4.9 – 5.3) | 5.1 (4.9 – 5.3) | 5.0 (4.8 – 5.2) |
Note: Values reported as median, interquartiles ranges.
Thank You Reading!
Please share any comments, feedback, relevant questions, or unrelated questions that you would like to see addressed in future blog posts.
Dr. Forey, Thanks for a very information post. I”m 46 yrs old with a1c 5.9 with LPIR score of 32. I did fasting insulin came out on the low side (1 μIU/mL). Suspected insulin resistance, but now not sure with low fasting insulin. Any thoughts?
I know this is not a medical advise, just wondering what your thoughts is as someone who has been researching in this area for sometime.
Thank you for your message. This does not represent medical advise or a response to your specific lab testing. An elevated Hemoglobin A1c can exist in both the presence and absence of insulin resistance. If there is an elevated HbA1c, a low fasting insulin is reassuring that insulin resistance may not be present. Another reassuring finding is an LPIR Score < 35, which also argues against insulin resistance. The remaining question at hand is average blood glucose monitoring, which can be achieved with continuous glucose monitoring. If values are persistently elevated, this could raise consideration for early type I diabetes, which can occur in the presence of low insulin and low C-peptide. Meanwhile, this occurs more often in individuals younger than age 45. A formal evaluation by a licensed healthcare professional would be reasonable if any concerns or lab abnormalities are present.
Follow up to my previous comments. I tested negative for all four antibody tests GAD65, IA-2, Insulin Antibody and ZnT8, but low c-peptide (0.74ng/ml) confirm the low fasting insulin test.